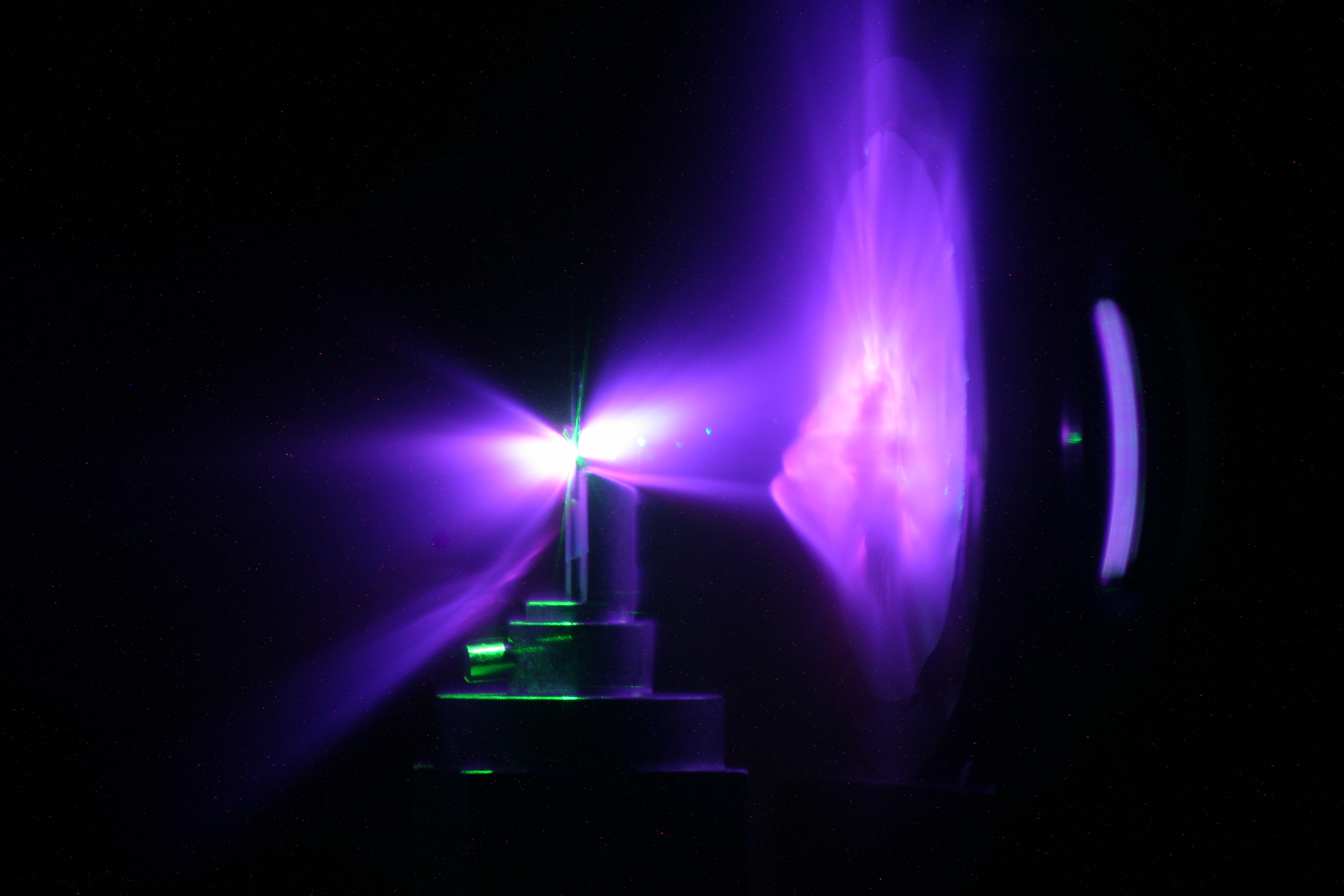
Plasma Physics is committed to scientific excellence in basic and applied research. Our breadth and depth in experimental thermonuclear plasma physics and associated high energy density systems, is applied to solving scientific and technical problems of importance to the nation and to the world.
We apply an extensive knowledge of plasma, atomic and laser-matter interaction physics, plasma chemistry, laser and pulsed-power technology, and complex diagnostics to study matter under extreme conditions in states ranging from plasma to condensed matter.
Research
Our research interests include
- Radiation hydrodynamics
- Shock properties
- X-ray, and gamma and neutron radiation production and detection
- Thermonuclear burn
- Radiation transport
- Opacity
- Neutron diagnostics and imaging
- High speed imaging and machine learning applications
- Inertial confinement fusion
- Magnetized fusion energy
- Magneto-inertial fusion
- Kinetic plasma effects
P-4’s staff uses x-ray and gamma-ray spectroscopy, ultrafast detectors, radiography, infrared imaging, magnetic probes, nuclear measurements, and advanced diagnostics and machine learning techniques to advance our research.
Teams
Plasma Physics includes scientists, engineers, technicians, and administrative support staff who team both within the group and externally to fulfill our missions. Our group includes a vibrant postdoctoral and graduate student community working on nuclear physics, fluid dynamics, astrophysics, and material science.
Radiation transport and material properties - Radiation transport, such as from the dense core of a star to the photosphere, is important in both astrophysics and high-energy-density-research. One of the most important aspects of properly understanding this complex system is how radiation is transported through and around materials in these extreme states. Material properties like x-ray opacities are governed by intricate atomic physics processes. This physics is being studied by brand new diagnostics in experiments at the National Ignition Facility and the Omega Laser Facility to elucidate how small deviations in measured x-ray opacities can have a huge impact on our understanding of how the sun works. X-ray opacities of materials at solar conditions and the complicated radiative transport processes that take place as radiation travels through and around a medium are being measured in experiments at the National Ignition Facility and the Omega Laser Facility.
Thermonuclear burn - Using advanced targets and diagnostic instruments, we study the way that inertial confinement fusion implosions generate thermonuclear yield. Using gamma rays, the burn duration and timing of the burn is measured and used to constrain simulations of the experiment. We improved the time resolution of the diagnostic to 0.01 billionths of a second and the measurements are starting to reveal the way the fuel assembles and burns. In other experiments, the effect of mixing of non-thermonuclear matter is measured and used to understand the interplay between burn and mixing. An additional source of mixing is the role that plasma kinetic effects play on moving capsule material into the burning fuel. This suite of experiments uses the Omega Laser Facility and the National Ignition Facility.
Implosion physics - Imploding a spherical capsule compresses the nuclear fuel and creates thermonuclear burn. One way to reach this state is to use two concentric capsules, known as a "double-shell." The outer capsule implodes a short distance at low velocity, strikes a higher atomic number (Z) capsule and causes it to implode. The higher-Z capsule reflects radiation from the fuel, keeping it at higher temperature, while the higher density of the shell keeps the fuel confined longer. P-4 is designing and fielding experiments at the National Ignition Facility (NIF) to create a volume ignition source of neutrons using this "doubleshell" burn concept. Experiments at NIF and the Omega Laser Facility examine features of the implosions, such as hydrodynamic instabilities, shape transfer, and engineering feature mitigation. Cylindrical implosions at both NIF and Omega test our understanding of hydrodynamic instabilities in a converging system. Contributing to to the understanding of these and high-energy density systems is investigation of utilization of machine learning techniques and high speed image analysis.
Diagnostic engineering - Our researchers design and build advanced x-rays and nuclear diagnostics for national and international customers. The team is designing and constructing an upgrade to the neutron imaging system at the National Ignition Facility (NIF) to capture tomographic images of the thermonuclear core of the plasma and to determine its properties. We are also responsible for the gamma reaction history diagnostic systems at NIF, which measure the thermonuclear burn rate and timing of the burn for high-yield implosions. P-4 designs and builds x-ray measurement systems that are used to determine the density of foams and other low-density materials used in high-energy-density targets. In addition, the team designs experiments for Los Alamos's proton radiography and plutonium facilities.
Magnetized plasmas - Research in magneto-inertial fusion includes topical areas of x-ray sources, collisional and collisionless magnetized shocks, field-reversed configuration confinement, and flow-dominated plasmas. On-site we use the Plasma Liner eXperiment to produce interactions of jets with other jets and background plasmas to study their interactions, the generation of x-rays, and the formation of plasma liners. Onsite we are building a Magnetized Shock Experiments aimed at producing x-ray sources. We aim to understand the basic physics of magnetized plasmas and support novel concepts for magnetic fusion. At off-site facilities, we collaborate on diagnostics systems for stellarators, tokamaks, and advanced light sources for radiation science experiments.
Facilities
Plasma Physics operates the following facilities.
- Magnetized Shock Experiment: Field-reversed configuration plasma devised for magnetized target fusion development
- Plasma Liner Experiment: Converging and colliding plasma jets for inertial confinement fusion and basic astrophysics
Plasma Physics supports laboratories such as x-ray calibration, target characterization, optical development and darkrooms, and engineering design.
Experiments at world-class facilities provide the drivers and instruments to develop and validate new models of important phenomena in plasma physics. These facilities include the:
- National Ignition Facility at Lawrence Livermore National Laboratory
- Omega Laser Facility at the University of Rochester
- Z Pulsed Power Facility at Sandia National Laboratories
- Wendelstein 7-X stellarator at the Max Planck Institute for Plasma Physics
- Linac Coherent Light Source, x-ray free electron laser at SLAC-Standford Linear Accelerator